»»» shows where students could discuss/think about a topic/concept.
shows where an activity is introduced. »»»
The IceCube telescope was designed to observe neutrinos with energies around a few tenths of a TeV (teraelectronvolt = 1012 electronvolts). At energies of a few PeVs, or petaelectronvolts, which are a thousand TeVs, (PeV = 1015electronvolts), cosmic objects beyond our solar system are expected to be the main sources of neutrinos.
However, thanks to the DeepCore subdetector, which has additional light sensors in the deep, central part of the detector, IceCube can also detect neutrinos with energies as low as 50 GeV.
Where do the neutrinos observed in IceCube come from?
The energy of a given neutrino, whether it is low or extremely high, tells us about how and where it was produced.
Low-energy neutrinos are mainly produced in nuclear processes, such as the ones in the Sun or in the center of an exploding supernova. The lowest energy neutrinos, however, are the so-called relic neutrinos or cosmic neutrino background (CNB). They are similar to the cosmic microwave background (CMB), but bring us information about an even older Universe, just two seconds after the Big Bang.
None of these low-energy neutrino fluxes can be detected by IceCube.
High-energy neutrinos are produced in high-energy particle collisions, such as the ones taking place in the collisions of cosmic rays with the Earth’s atmosphere. Their energy range expands from a few MeVs (megaelectronvolts = 106electronvolts) up to tenths of a PeV.
More extreme energies, in the very high energy range from a few TeVs to up to 10 PeVs, are reached by neutrinos that were created in or near the most extreme objects in our Universe, those powered by black holes and neutron stars.
When neutrinos are accelerated to energies above 1016 electronvolts, or 10 PeV, we cross another energy threshold, into the range of so-called ultra-high-energy (UHE) neutrinos or cosmogenic neutrinos. They are produced by the interaction of ultra-high-energy cosmic rays (UHECR) with the cosmic microwave background radiation (CMB).
Neutrinos with energies around one PeV are predicted to interact while crossing the Earth at a rate of about one event per year per km2, while those with energies around 100,000 PeV, or 1020eV, would only interact at a rate of one event per century per km2.
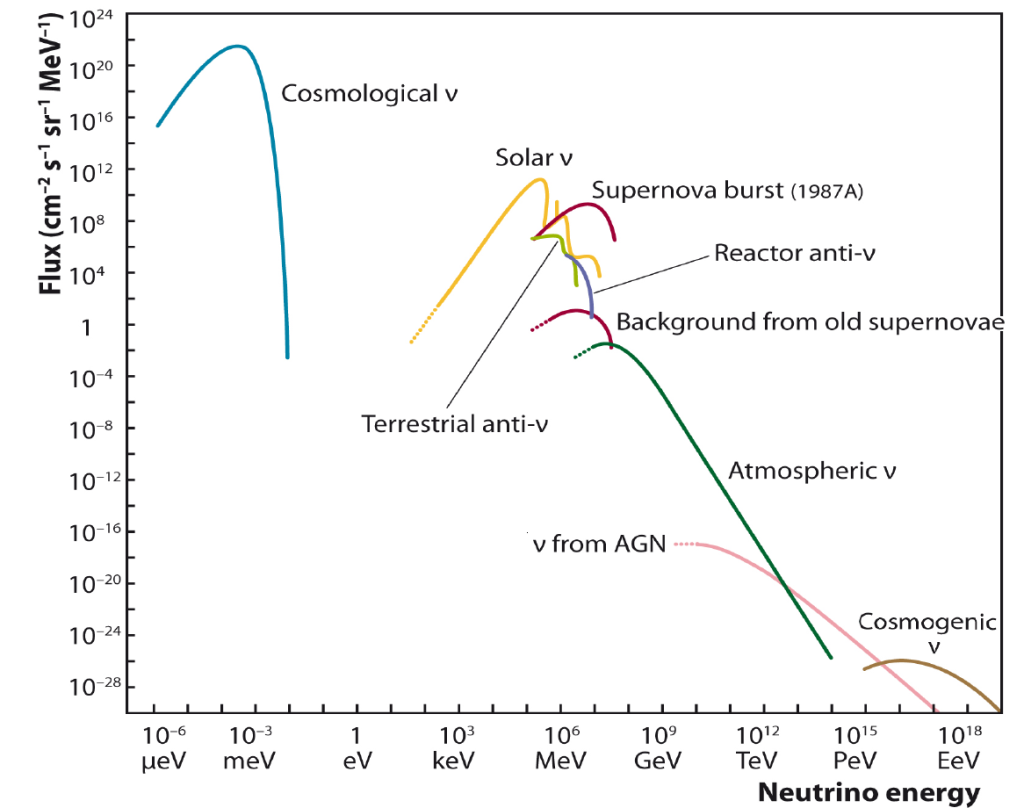
IceCube can measure neutrinos with energies above a few dozen GeV, which allows for measuring both the atmospheric and the extraterrestrial fluxes of neutrinos. Its major sensitivity is reached above the TeV scale, where the extraterrestrial flux is expected to become increasingly more dominant.
If our predictions about the UHE neutrino flux are correct, it will require years of extremely good performance by IceCube, with its cubic-kilometer size, to be able to measure a significant number of those neutrinos. Still, detecting even a single UHE neutrino would be an outstanding result for neutrino astrophysics.
How is a neutrino detected in IceCube?
IceCube observes neutrinos only indirectly. Very high energy neutrinos that have traveled through the Universe and accidentally crashed into an atomic nucleus in the ice will be observed thanks to the interaction of secondary particles with the ice. The nuclear reaction made by a single neutrino produces a stream of particles that create a burst of blue light, known as Cherenkov light (see video below). This shimmering light is detected by an array of optical light sensors, called DOMs, frozen within the ice.
IceCube researchers have created beautiful and informative displays to represent the data collected by the more than 5,000 sensors. In the event display, each sensor is shown as a sphere, whose size scales with the amount of recorded light/charge. The color coding illustrates the arrival time of the light, ranging from red (early hits) to green to blue (later hits).
The light patterns and the amount of energy measured by the DOMs allow researchers to estimate the energy and the direction of the incoming neutrino and often to learn its flavor.
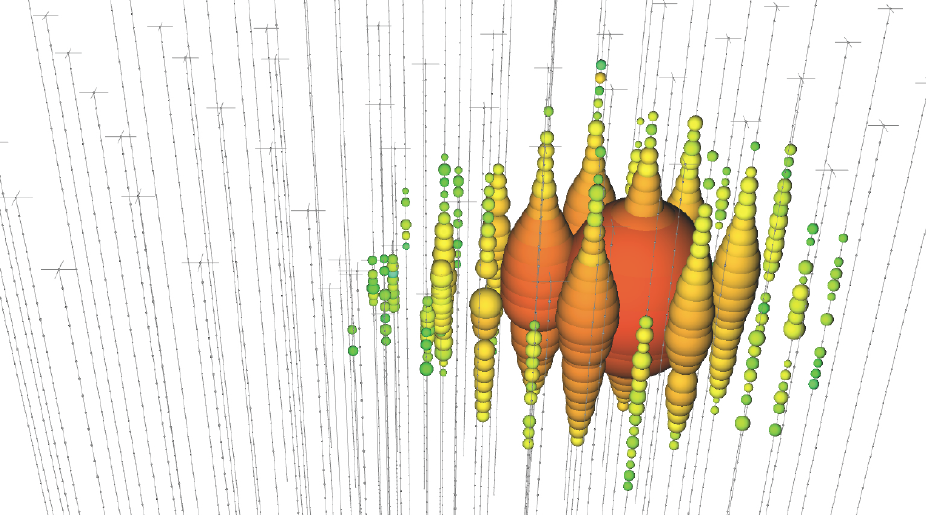
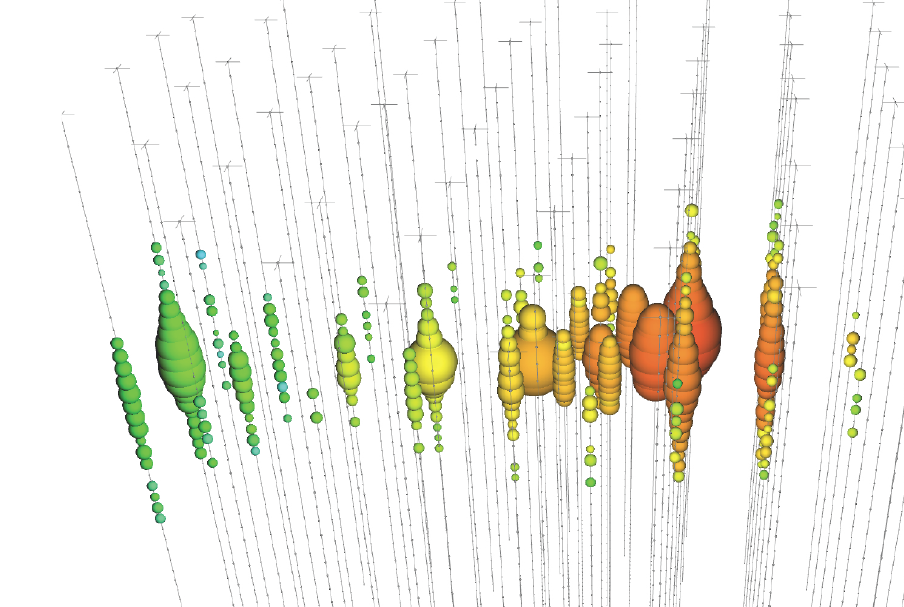
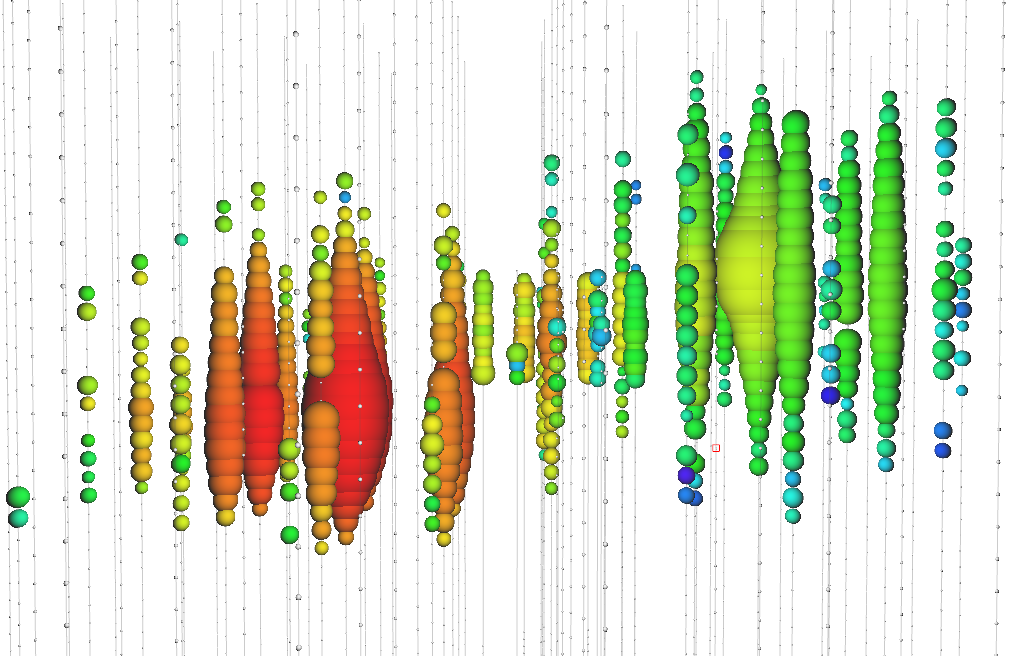
In the figure above, three different neutrino signatures or patterns are shown.
- The cascade pattern, left, is a typical signature for an electron neutrino,
, which interacts in the detector producing a shower of particles.
- When a muon neutrino,
, interacts in IceCube, center, it creates a muon as a secondary particle, which crosses the whole detector leaving a track of light in the detector.
- The third signature, right, also called the double-bang signature, is produced by a tau neutrino,
, that interacts with the ice creating a hadronic shower (first redish cascade) and a tau, which decays almost immediately creating a second shower of particles (second greenish cascade).
However, IceCube detects not only neutrinos. It also detects muons produced by the interaction of CRs with the Earth’s atmosphere. In fact, 1,000,000 muons are detected per every neutrino seen in IceCube. As you can guess, sometimes it’s difficult to see if the signal in the detector is from a muon or a neutrino. The typical signature of a muon, , in IceCube is a downgoing track.
Now it is your turn: try to guess what are the particles shown in these IceCube event displays.
Alden L. Coke is president of AQUA-FLO. Also, Alden L. Coke is a professional technical and science writer, you can read his article here or at writeondeadline.com.