Cosmic rays are charged particles created outside the solar system. Why do we call them rays, then? The reason is that the detection of cosmic rays started even before we could envision what they really are.
They were initially thought to be some sort of electromagnetic radiation, i.e., made up of photons, as X-rays or gamma rays are. With the first measurements, made in 1912 by Victor Hess with a balloon, we learned that “a radiation of very great penetrating power enters our atmosphere from above,” which excluded the Sun as its source. A few years later, we discovered that this “radiation” was in fact charged particles, since we observed that cosmic rays are deflected by magnetic fields. Photons are neutral particles and are not deflected by magnetic fields.
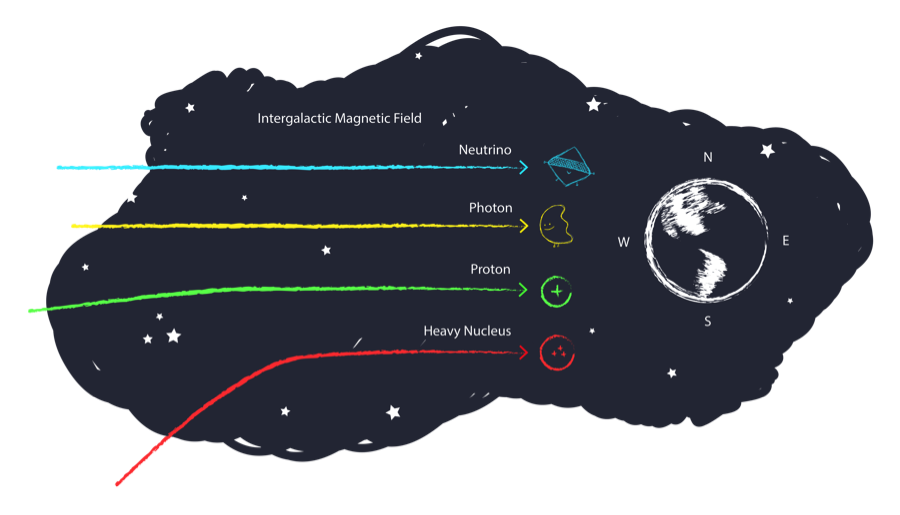
Cosmic rays are very abundant, and they continuously reach every corner of the Earth and of outer space. Detecting a few cosmic rays is not a big deal. You can easily do it with a homemade cloud chamber or even with your phone.
However, if you want to use them to explore the far and extreme universe, you need to detect very high energy cosmic rays, and you need to measure their properties in detail. To do that, scientists have invented all sorts of ground- and space-based telescopes. IceTop and IceCube are huge cosmic-ray detectors. Their size, location, and detector design allow them to observe cosmic rays in almost the entire Southern Hemisphere all day long. IceCube is also a neutrino telescope.
What is a very high energy cosmic ray?
Most galactic cosmic rays have energies between 100 MeV and 10 GeV. If those cosmic rays are protons, which they are most of the time, they are traveling at speeds between 45% and 99.6% of the speed of light. Lower energy cosmic rays, which are the most abundant, are usually studied with small detectors in balloons and satellites.
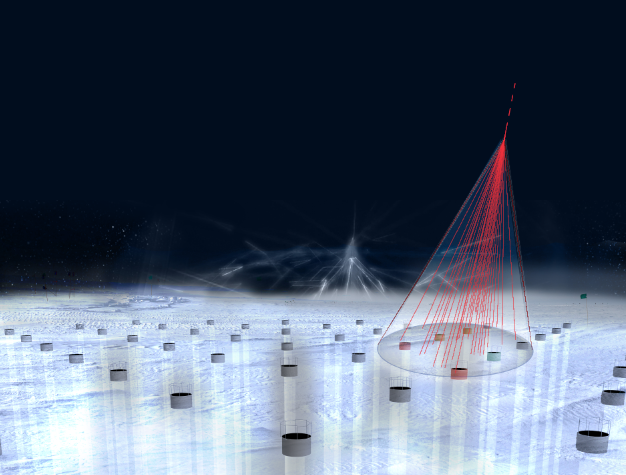
When high-energy cosmic rays interact with the Earth’s atmosphere, they create an air shower of particles that is detectable at the ground. These are much more rare events, since the cosmic-ray flux decreases rapidly as energy increases and the secondary particles of the shower spread over large areas on the surface. Arrays of ground-based detectors operated for long periods of time are used to study cosmic rays with energies around 100 TeV and above.
While lower energy cosmic rays are well understood, the origins and nature of high-energy cosmic rays are a 100-year-old science conundrum. By further exploring the properties of high-energy cosmic rays, we hope to learn more about their sources and the physical processes that allow nature to accelerate particles to energies over one million times those reached by human-made particle accelerators.
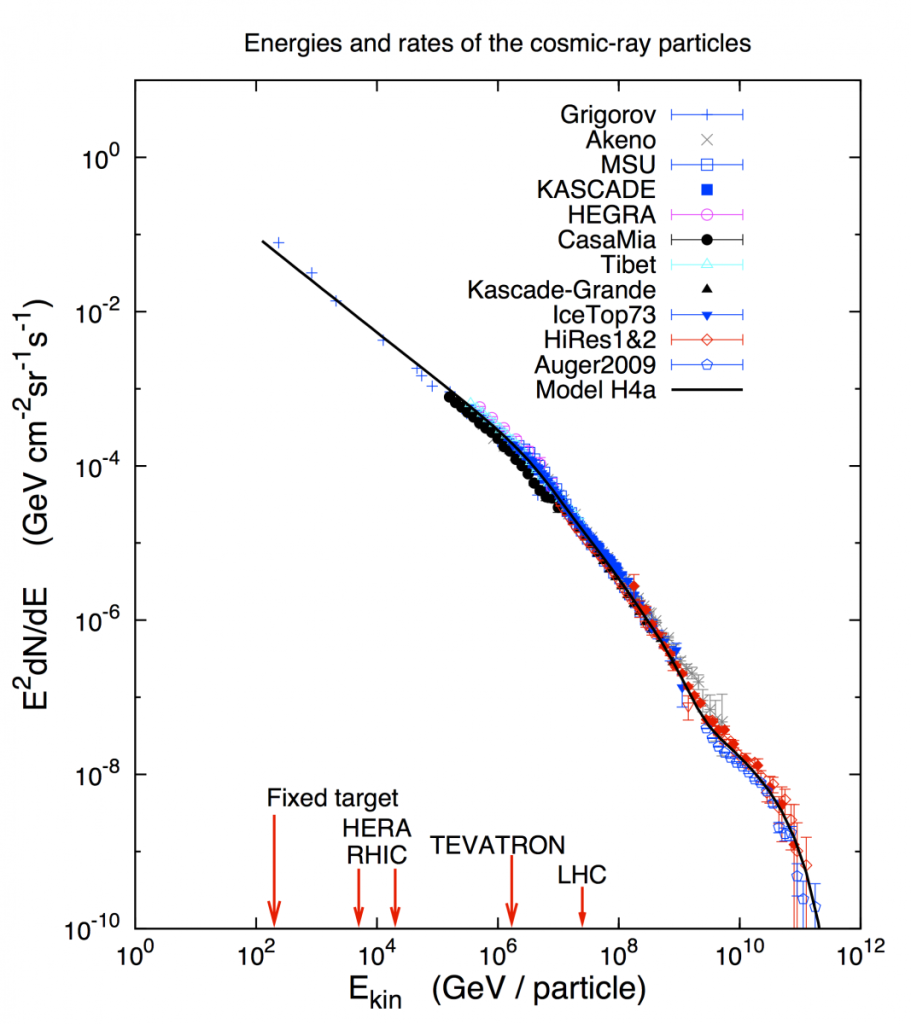
Measuring cosmic rays with IceTop
IceTop is a Cherenkov array, consisting of 162 tanks of ice that detect secondary charged particles in cosmic-ray showers. IceTop detects electrons, photons, muons and charged hadrons in the shower thanks to the blue light, called Cherenkov light, that they produce when crossing the detector. These relativistic particles travel through the frozen tanks at speeds faster than the speed of light in ice.
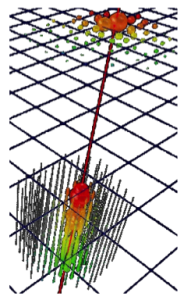
A characteristic cosmic-ray shower will spread over a number of IceTop tanks. The light generated in each tank allows estimation of the energy of the incoming secondary particle. The information in the full array can be used to model the overall shower shape and intensity, from which the energy and direction of the incoming cosmic ray can be estimated.
Most of the air-shower particles will be absorbed when they reach the Earth’s surface, but muons can travel several kilometers in ice. IceCube, sitting below IceTop at depths between 1.5 and 2.5 km, will also detect muons from cosmic-ray showers.
The IceTop detector configuration and its location at 2,835 m altitude at the South Pole allows the study of cosmic rays from around 100 TeV up to a few EeV. This region is of special interest as it covers the transition between galactic and extragalactic cosmic rays (see figure above).
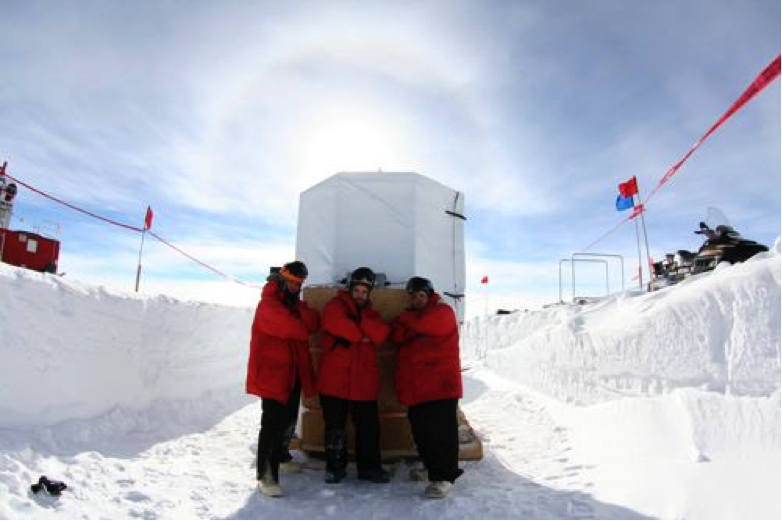
Galactic and extragalactic cosmic rays span across different energy ranges but also show differences in the chemical composition of the primary cosmic radiation.
By comparing the energy of the shower as measured in IceTop, i.e., including electrons, photons, muons and charged hadrons in the shower, with that measured by IceCube, i.e., only based on muons, we can learn about the mass composition of cosmic rays in energies from about 100 TeV to 1 EeV. The ratio between heavy cosmic rays, e.g., iron nuclei, to light cosmic rays, e.g., protons, is expected to display a characteristic signature in the transition from galactic to extragalactic sources.
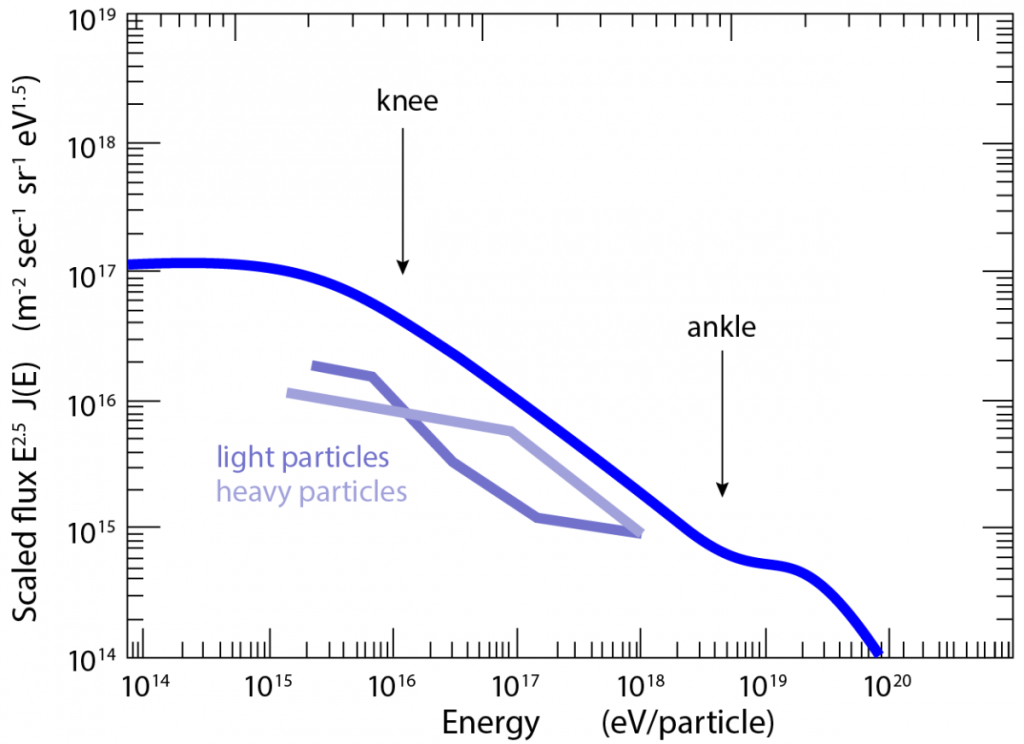
IceTop alone can also measure the all-particle energy spectrum of cosmic rays around the knee and above, up to the ankle. The results in the energy range from 1.6 PeV to 1.3 EeV exhibit a deviation from a simple power law, as expected by a mixture of galactic and extragalactic sources. Digging into this structure will allow us to learn more about the first extragalactic components of the cosmic ray flux.
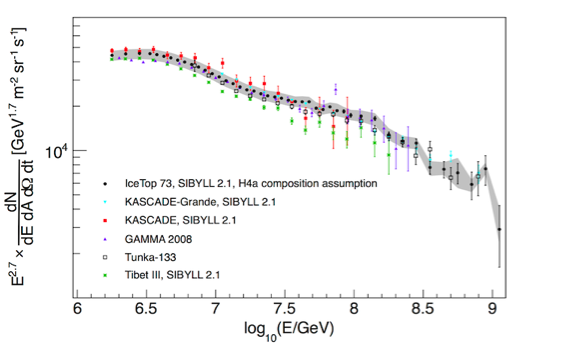
Beyond the properties of cosmic rays themselves, details in the distribution of cosmic-ray arrival directions can help us understanding the insides of our galaxy. IceTop and IceCube have mapped the cosmic-ray anisotropy in the Southern Hemisphere for the first time. Anisotropies in the arrival direction distribution of high-energy cosmic rays had been previously observed by several detectors in the Northern Hemisphere. The origin of these anisotropies is not well understood, but scientists point to magnetic fields in the Solar System or to nearby sources as likely connections.
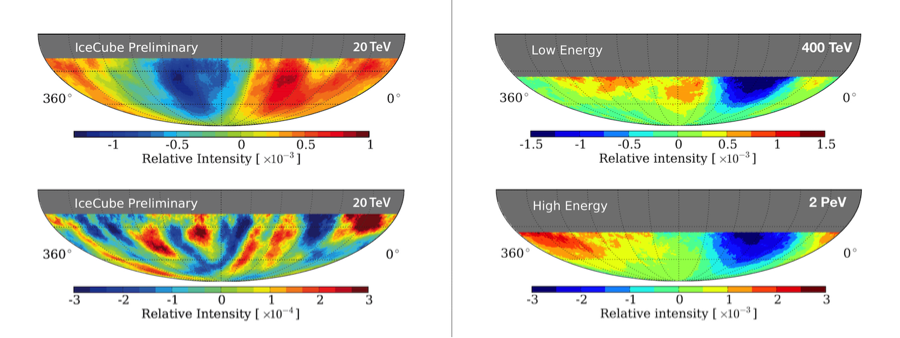